Is There an Optimal Dosage for Veterinary Shockwave?
Determining the optimal dose for veterinary use of therapeutic shockwave is a complex task that lacks a straightforward solution. Several parameters come into play when applying therapeutic shockwave treatment, including the number of pulses administered, the intensity of energy delivered (expressed as EFD in mJ/mm2), the frequency at which the pulses are applied (in Hz), the total number of treatments, and the time period between treatments. Achieving the appropriate dosage for treating animals necessitates a comprehensive understanding of the physics and mechanism of action of shockwaves, a correct diagnosis, sound anatomical knowledge of the patient, and the ability to interpret the animal's subtle reactions during the administration of the treatment.
Shockwaves exert their influence on tissue by engaging in mechanotransduction, a process wherein acoustic waves traverse through the tissue, introducing physical forces such as pressure. These forces, in turn, trigger a cascade of biochemical changes that impact the overall health of the tissue. These changes encompass various vital aspects, including cell growth, cell differentiation, cell migration, protein synthesis, physiological apoptosis, and tissue necrosis1. Several cell types are particularly sensitive to mechanical stimuli, including tenocytes, fibroblasts, osteocytes, chondrocytes, and endothelial cells. At the molecular level, these biochemical changes ultimately modulate the gene expression of cells, thereby eliciting specific reactions within the tissue2.
Shockwave therapy has been the subject of extensive research, uncovering a range of effects it produces. These effects include the release of nitric oxide, IGF, TGFbeta, and VEGFgamma, as well as the stimulation of bone metabolism, lubricin production, and activation of stem cells3-8.
Determining the appropriate energy level to stimulate in vivo mechanotransduction is a challenging task. Energy flux density (EFD) measured in mJ/mm2 or peak pulse energy in MPa are values commonly used to quantify the local energy generated and emitted by shockwave devices, serving as initial reference points.
Currently, EFD is the predominant value reported in literature and utilized by ELvationUSA-Vet in the PiezoWave2-Vet manuals to represent energy. However, it's important to note that EFD only reflects the assumed energy density in mJ/mm2 at the center of the focal zone and does not correspond to the total energy output, which includes both the focal area and a larger surrounding area with lower pressure. During the design and manufacturing of shockwave devices, the overall energy output is measured in a controlled laboratory environment using an in vitro water-based system and a hydrophone. Therefore, all energy outputs and device settings provided are based on these controlled conditions.
Nevertheless, when acoustic waves travel through tissues with varying acoustic impedances and at different speeds, the energy released at tissue interfaces undergoes attenuation or enhancement through processes such as reflection, scattering, and transmission 9,10. This poses a challenge in determining the required dose for initiating mechanotransduction since the EFD measured in vitro cannot directly correlate to the amount of energy present at specific tissue sites. Additionally, comparing different shockwave systems becomes difficult due to variations in focal zone volume and slight changes in focal zones at different energy levels, specific to each therapy source or trode9.
Various studies have been conducted to compare the biological effects and pain levels associated with different energy levels in shockwave therapy. In veterinary medicine, clinical studies have utilized slightly varied protocols, but most commonly employed electrohydraulic shockwave generators. These protocols typically involve 750-1000 pulses, an energy flux density (EFD) of approximately 0.15 mJ/mm2, and a treatment series consisting of 2-4 sessions every 2-4 weeks 11-18.
Recently published case studies focused on shoulder tendon and muscle injuries utilizing the PiezoWave device and demonstrated tissue healing with four treatments at energy levels ranging from 0.032-0.064 mJ/mm2, administered once or twice per week19. These energy levels are lower than those previously reported in veterinary studies. However, there is a human study on shockwave therapy for knee osteoarthritis where an energy level of 0.04 mJ/mm2 provided equivalent pain relief compared to 0.093 mJ/mm2. The treatment protocol in this study involved 1000 pulses administered weekly for 3 weeks, utilizing an electromagnetic generator20.
Other human studies comparing shockwave therapy doses suggest that lower energy levels are preferable for pain relief and healing in soft tissue conditions 21-23. Conversely, higher energy levels have been reported to be effective for repairing non-healing bone compared to fresh fractures24. When it comes to calcification in tendons, higher energy levels seem to be required compared to non-calcified tendinopathies25.
Several variables can influence the amount of energy needed to induce a physiological response, including differences in tissue acoustic impedance, the metabolism of various tissue types, and the ability of the energy to reach different structures within the body.
In veterinary medicine, ElvationUSA has observed a trend suggesting that low-energy shockwave therapy, specifically at or below 0.1 mJ/mm2, with a total of 3-6 treatments, is not only well-tolerated but also less likely to cause post-treatment discomfort while still effectively promoting healing in soft tissue injuries. Accurate targeting of the energy to the intended tissue is of utmost importance, as improper targeting can lead to variations in treatment outcomes.
Despite the variations in energy levels described in different protocols, it is universally agreed upon that therapeutic shockwave therapy is safe. The existence of diverse protocols yielding successful outcomes indicates that therapeutic shockwave can be applied in various ways, and thus there is no definitive right or wrong protocol. The key to achieving success lies in comprehending the underlying principles and determining the appropriate application site for the energy.
In devising well-informed shockwave treatment plans, it is crucial to consider not only the energy employed but also the complete treatment protocol. This includes factors such as the specific type of shockwave utilized, the number of pulses administered, the depth of penetration, the frequency of treatments, and concurrent therapies being employed simultaneously. By considering these elements collectively, a more informed and effective approach to shockwave therapy can be developed.
A few of the many unanswered questions include:
- How much energy is needed to initiate mechanical transduction in different types of tissue?
- Is more energy better?
- How many treatments are needed and how should they be spaced for best tissue healing?
- Is there a way to standardize energy output between different therapeutic shockwave generators?
- Is excess energy to surrounding health tissue beneficial, detrimental, or neither?
References
- Wang J., Li B. (2010) Mechanics rules cell biology. Sports Medicine, Arthroscopy, Rehabilitation, Therapy & Technology. 2: 16. doi: 10.1186/1758-2555-2-16.
- d'Agostino, M. C., Craig, K., Tibalt, E., & Respizzi, S. (2015). Shock wave as biological therapeutic tool: From mechanical stimulation to recovery and healing, through mechanotransduction. International journal of surgery, 24, 147-153.
- Yan X, et al. (2008) Improvement of blood flow, expression of nitric oxide, and vascular endothelial growth factor by low-energy shockwave therapy in random-pattern skin flap mode. Plastic Surgery.61(6):646-53. doi: 10.1097/SAP.0b013e318172ba1f.
- Atesok K., et al. (2014) Augmention of tendon-to-bone-Healing. Current Concepts Review. Journal of Bone Joint Surgery of America. 96(6):513-21. doi: 10.2106/JBJS.M.00009.
- Berta, L., et al. (2009)Extracorporeal shock waves enhance normal fibroblast proliferation in vitro and activate mRNA expression for TGF-•1 and for collagen types I and III. Acta Orthopaedica. 80 (5): 612-617.
- Chen Y J, et al. (2004) Extracorporeal shock waves promote healing of collagenase-induced Achilles tendinitis and increase TGF-β1 and IGF-I expression. Journal of Orthopaedic Research.22(4)854-861. doi.org/10.1016/j.orthres.2003.10.013
- Zhang D., et al. (2011) Extracorporeal shockwave-induced expression of lubricin in tendons and septa. Cell Tissue Research.346:255-262. Doi: 10.1007/s00441-011-1258-7.
- Neuland, H. G., Schmidt, A. (2006) Induction of adult (tissue-specific) mesenchymal stem cells through extracorporeal shock waves to regenerate muscoskeletal Tissue. Orthopaedische Praxis. 42: 4.
- ISMST, DIGEST Guidelines for Extracorporeal Shockwave, available on line (the energy blog has a link to this)
- 10.Ogden, J. A., Tóth-Kischkat, A., & Schultheiss, R. (2001). Principles of shock wave therapy. Clinical Orthopaedics and Related Research (1976-2007), 387, 8-17.
- Becker, W., Kowaleski, M. P., McCarthy, R. J., & Blake, C. A. (2015). Extracorporeal shockwave therapy for shoulder lameness in dogs. Journal of the American Animal Hospital Association, 51(1), 15-19.
- Leeman, J. J., Shaw, K. K., Mison, M. B., Perry, J. A., Carr, A., & Shultz, R. (2016). Extracorporeal shockwave therapy and therapeutic exercise for supraspinatus and biceps tendinopathies in 29 dogs. Veterinary Record, 179(15), 385-385.
- Barnes, K., Faludi, A., Takawira, C., Aulakh, K., Rademacher, N., Liu, C. C., & Lopez, M. J. (2019). Extracorporeal shock wave therapy improves short‐term limb use after canine tibial plateau leveling osteotomy. Veterinary Surgery, 48(8), 1382-1390.
- Kieves, N. R., MacKay, C. S., Adducci, K., Rao, S., Goh, C., Palmer, R. H., & Duerr, F. M. (2015). High energy focused shock wave therapy accelerates bone healing. Veterinary and Comparative Orthopaedics and Traumatology, 28(06), 425-432.
- Trager, L. R., Funk, R. A., Clapp, K. S., Dahlgren, L. A., Werre, S. R., Hodgson, D. R., & Pleasant, R. S. (2020). Extracorporeal shockwave therapy raises mechanical nociceptive threshold in horses with thoracolumbar pain. Equine veterinary journal, 52(2), 250-257.
- Bischofberger, A. S., Ringer, S. K., Geyer, H., Imboden, I., Ueltschi, G., & Lischer, C. J. (2006). 15. Histomorphologic evaluation of extracorporeal shock wave therapy of the fourth metatarsal bone and the origin of the suspensory ligament in horses without lameness. American journal of veterinary research, 67(4), 577-582.
- McClure, S. R., VanSickle, D., Evans, R., Reinertson, E. L., & Moran, L. (2004). The effects of extracorporeal shock-wave therapy on the ultrasonographic and histologic appearance of collagenase-induced equine forelimb suspensory ligament desmitis. Ultrasound in medicine & biology, 30(4), 461-467.
- Giunta, K., Donnell, J. R., Donnell, A. D., & Frisbie, D. D. (2019). Prospective randomized comparison of platelet rich plasma to extracorporeal shockwave therapy for treatment of proximal suspensory pain in western performance horses. Research in veterinary science, 126, 38-44.
- Owen, H. (2022). Case study: Treating infraspinatus and supraspinatus trigger points and supraspinatus tendinopathy utilizing piezoelectric shockwave. Frontiers in Veterinary Science, 9.
- Kim, J. H., Kim, J. Y., Choi, C. M., Lee, J. K., Kee, H. S., Jung, K. I., & Yoon, S. R. (2015). The dose-related effects of extracorporeal shock wave therapy for knee osteoarthritis. Annals of Rehabilitation Medicine, 39(4), 616-623.
- Taheri, P., Emadi, M., & Poorghasemian, J. (2017). Comparison the effect of extra corporeal shockwave therapy with low dosage versus high dosage in treatment of the patients with lateral epicondylitis. Advanced biomedical research, 6.
- Yang, Y. J., Lee, S. J., & Matthew, C. (2014). Pressure pain threshold and visual analogue scale changes in the high and low energy extracorporeal shock wave. Physical therapy rehabilitation science, 3(2), 142-147.
- Park, K. D., Lee, W. Y., Park, M. H., Ahn, J. K., & Park, Y. (2018). High-versus low-energy extracorporeal shock-wave therapy for myofascial pain syndrome of upper trapezius: A prospective randomized single blinded pilot study. Medicine, 97(28).
- Moretti, B., Notarnicola, A., Moretti, L., Patella, S., Tatò, I., & Patella, V. (2009). Bone healing induced by ESWT. Clinical cases in mineral and bone metabolism, 6(2), 155.
- Bannuru, R. R., Flavin, N. E., Vaysbrot, E., Harvey, W., & McAlindon, T. (2014). High-energy extracorporeal shock-wave therapy for treating chronic calcific tendinitis of the shoulder: a systematic review. Annals of internal medicine, 160(8), 542-549.
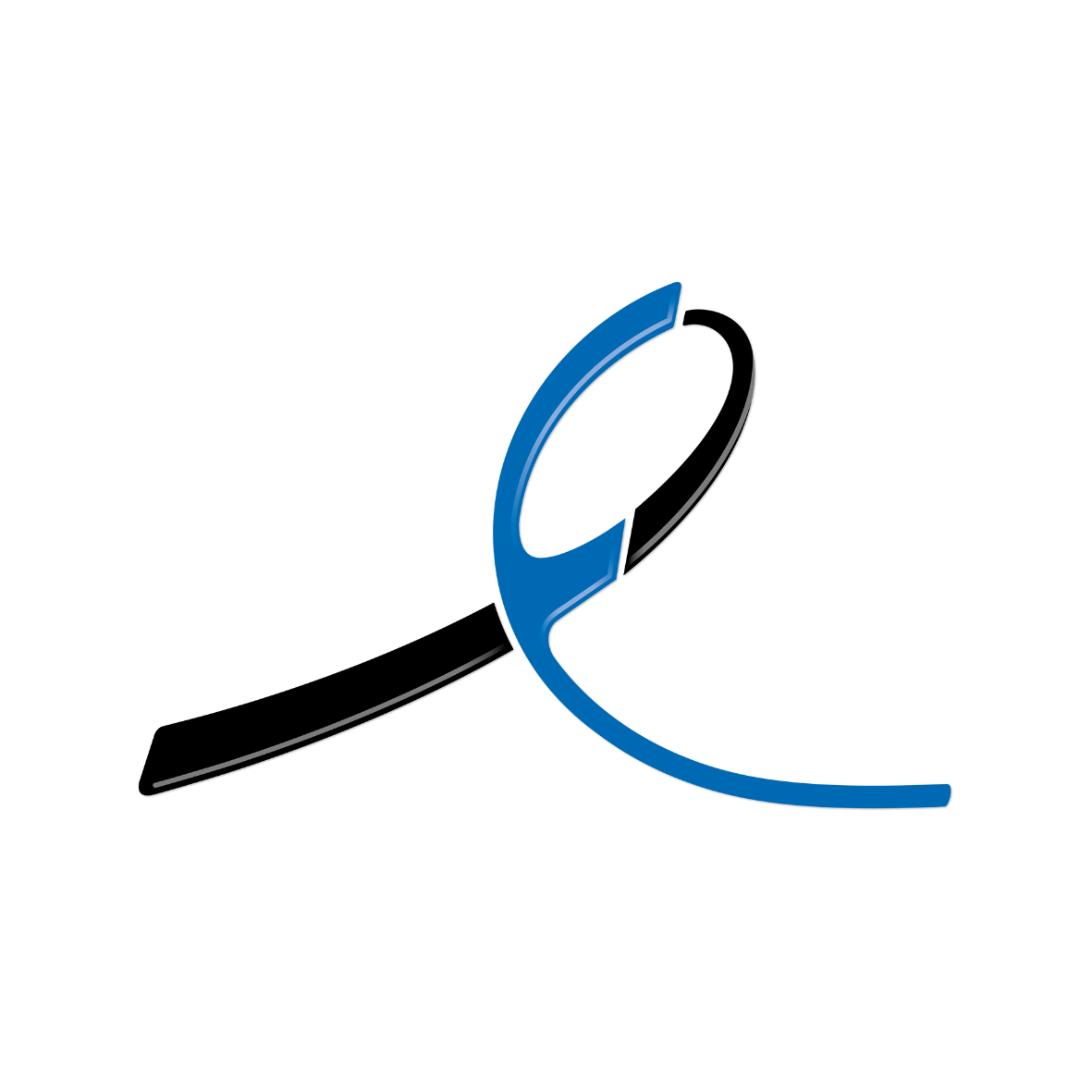
ELvation Marketing Team
Combining sales and flexible customer support with many years’ in-depth knowledge of medical equipment we offer customized solutions to create value with long-term investments and medical supplies. ELvation’s strength lies in its ability to combine the apparently contradictory needs of improving the standards of patient care by providing high-quality medical technology and good corporate profitability. We have a special partnership with Richard Wolf GmbH as their long-term authorized Sales & Service Team for piezo shockwave systems.