Shockwave Therapy in the Spinal Area - a Brief Literature Review
Explore the growing body of research on the application of shockwave therapy in the spinal area with this informative blog post. Delve into the science behind this non-invasive treatment, the conditions it can address, and the evidence supporting its efficacy. Stay informed on the latest advancements in veterinary medicine and how shockwave therapy is making a difference in spinal care for animals.
The use of shockwave directly over the spinal cord was listed as a contraindication by the ISMST (International Society for Medical Shockwave Treatment) in 2016 1. The ISMST guidelines were updated in 2021 and now include the spine in the treatment area as a “relative contraindication” and neurologic pathologies of spasticity and polyneuropathy as “expert indications” 2. This is just one example of how research and clinical experience on the use of shockwaves for neurological conditions continue to evolve. With the ongoing new findings in neurologic research, ElvationUSA has encouraged the use of the PiezoWave 2 Vet for treating both the paraspinal muscles and peripheral nerves while still advising the avoidance of the placement of the focal zone directly on the spinal cord. As shockwave usage evolves, a short literature review provides current and historical context for the use of shockwaves around the spine.
Effective orthopedic shockwave treatments evolved from their urologic applications for the treatment of calcaneal stones (lithotripsy) where high-energy pressure waves pulverize stones 3.
Over the years with a better understanding of the therapeutic mechanisms of actions (mechanical transduction), the trend has been to use lower energy 4. In 1998 using a rabbit Achilles tendon and an electromagnetic shockwave generator the dose of 1000 pulses at 0.60 mj/mm2 resulted in areas of necrosis in the tendon. Whereas, with 0.28 mj/mm2 there was no direct tendon damage but there was an increase in inflammatory reactions for 7 days post-treatment 5. This documentation of tissue damage helped to define high-energy shockwave (0.60 mj/mm2 and above) as a concern when using shockwaves for musculoskeletal therapy (Rompe 1988). This and other studies have led to a useful definition of high (greater than 0.6 mj/mm2, medium (between 0.2 mj/mm2 and 0.6) and low energy (often less than 0.2 mj/mm2) parameters to help guide treatment applications.
In 2007, the first study to address the dose-dependent effects of shockwave on the spinal cord used rabbits that had an L4 laminectomy seven days prior to electrohydraulic shockwave therapy. Different doses were used including; low energy dose defined as 2000 shockwave pulses at 0.18 mj/mm2, and high energy at 2000 pulses at 0.62 mj/mm2.
None of the treated rabbits displayed weakness or paralysis in the hind limbs. However, microscopic post-mortem examination of the spinal cord showed mild but dose-dependent myelin damage and the pathology of the spinal cord in the high-energy group showed a decrease in the number of neurons 6. Other studies have also suggested high-energy shockwaves may damage the spinal cord, the peripheral nerves, and the brain 7,8,9.
However, low-energy shockwave has been shown to have many positive neuronal effects. Low energy shockwave caused improved function in both peripheral nerve injuries and stroke patients 10,11. It also has been shown to increase VEGF expression and lower secondary neural tissue after spinal cord injury 12,13. BDNF (brain-derived neurotrophic factor) is suggested to have a therapeutic effect on spinal cord injuries and, in a rat model, has been shown to be upregulated by the low-energy shockwave and to reduce both functional impairment and damage to neuronal tissue 14. A 2022 study using a rat SCI model and low-energy shockwave demonstrated functional improvement but not morphological changes 14. However, significant changes in different miRNA species were identified and supplied insight into mechanisms of neuroplasticity and targets for therapeutic intervention 15. The protocol of a human clinical trial using low-energy shockwave to treat both sensory impairment and spasticity after spinal cord injury was published in 2022 16. Finally, neuronal stem cells have been shown to play an important role in both the central and peripheral nervous system and neuronal stem cell proliferation is promoted by low-energy shockwave 17,18.
References
1.(2016) About ESWT. The International Society for Medical Shockwave Treatment.
2. Introduction and prerequisites and minimal standards of performing ESWT.
3. (2020) Shockwave History. The International Society for Medical Shockwave Treatment.
4. d'Agostino, M. C., et al. "Shock wave as biological therapeutic tool: From mechanical stimulation to recovery and healing, through mechanotransduction." International journal of surgery 24 (2015): 147-153.
5. Rompe, J. D., et al. "Dose-related effects of shock waves on rabbit tendo Achillis: a sonographic and histological study." The Journal of bone and joint surgery. British volume 80.3 (1998): 546-552.
6. Lee, Tao-Chen, et al. "Vulnerability of the spinal cord to injury from extracorporeal shock waves in rabbits." Journal of Clinical Neuroscience 14.9 (2007): 873-878.
7. Karatas, A., et al. "The effect of extracorporeal shock wave lithotripsy on the rat spinal cord." Spinal cord 46.9 (2008): 627-632.
8. Wang, Ching-Jen, et al. "Pathomechanism of shock wave injuries on femoral artery, vein and nerve: an experimental study in dogs." Injury 33.5 (2002): 439-446.
9. Nakagawa, Atsuhiro, et al. "Application of shock waves as a treatment modality in the vicinity of the brain and skull." Journal of neurosurgery 99.1 (2003): 156-162.
10. Hausner, Thomas, et al. "Improved rate of peripheral nerve regeneration induced by extracorporeal shock wave treatment in the rat." Experimental neurology 236.2 (2012): 363-370.
11. Lee, Jung-Ho, Youn-Bum Sung, and Sang-Hun Jang. "Nerve growth factor expression in stroke induced rats after shock wave." Journal of Physical Therapy Science 28.12 (2016): 3451-3453.
12. Yamaya, Seiji, et al. "Low-energy extracorporeal shock wave therapy promotes vascular endothelial growth factor expression and improves locomotor recovery after spinal cord injury." Journal of neurosurgery 121.6 (2014): 1514-1525.
13. Yahata, Kenichiro, et al. "Low-energy extracorporeal shock wave therapy for promotion of vascular endothelial growth factor expression and angiogenesis and improvement of locomotor and sensory functions after spinal cord injury." Journal of Neurosurgery: Spine 25.6 (2016): 745-755.
14. Matsuda, Michiharu, et al. "Low-energy extracorporeal shock wave therapy promotes BDNF expression and improves functional recovery after spinal cord injury in rats." Experimental Neurology 328 (2020): 113251.
15. Ashmwe, Mohamed, et al. "Effects of Extracorporeal Shockwave Therapy on Functional Recovery and Circulating miR-375 and miR-382-5p after Subacute and Chronic Spinal Cord Contusion Injury in Rats." Biomedicines 10.7 (2022): 1630.
16. Leister, Iris, et al. "The effect of extracorporeal shock wave therapy in acute traumatic spinal cord injury on motor and sensory function within 6 months post-injury: a study protocol for a two-arm three-stage adaptive, prospective, multi-center, randomized, blinded, placebo-controlled clinical trial." Trials 23.1 (2022): 1-13.
17. Kisoh, Keishi, et al. "Possible involvement of PI3-K/Akt-dependent GSK-3β signaling in proliferation of neural progenitor cells after hypoxic exposure." Molecular Neurobiology 56.3 (2019): 1946-1956.
18. Richard, Seidu A., and Marian Sackey. "Elucidating the Pivotal Neuroimmunomodulation of Stem Cells in Spinal Cord Injury Repair." Stem Cells International 2021 (2021).
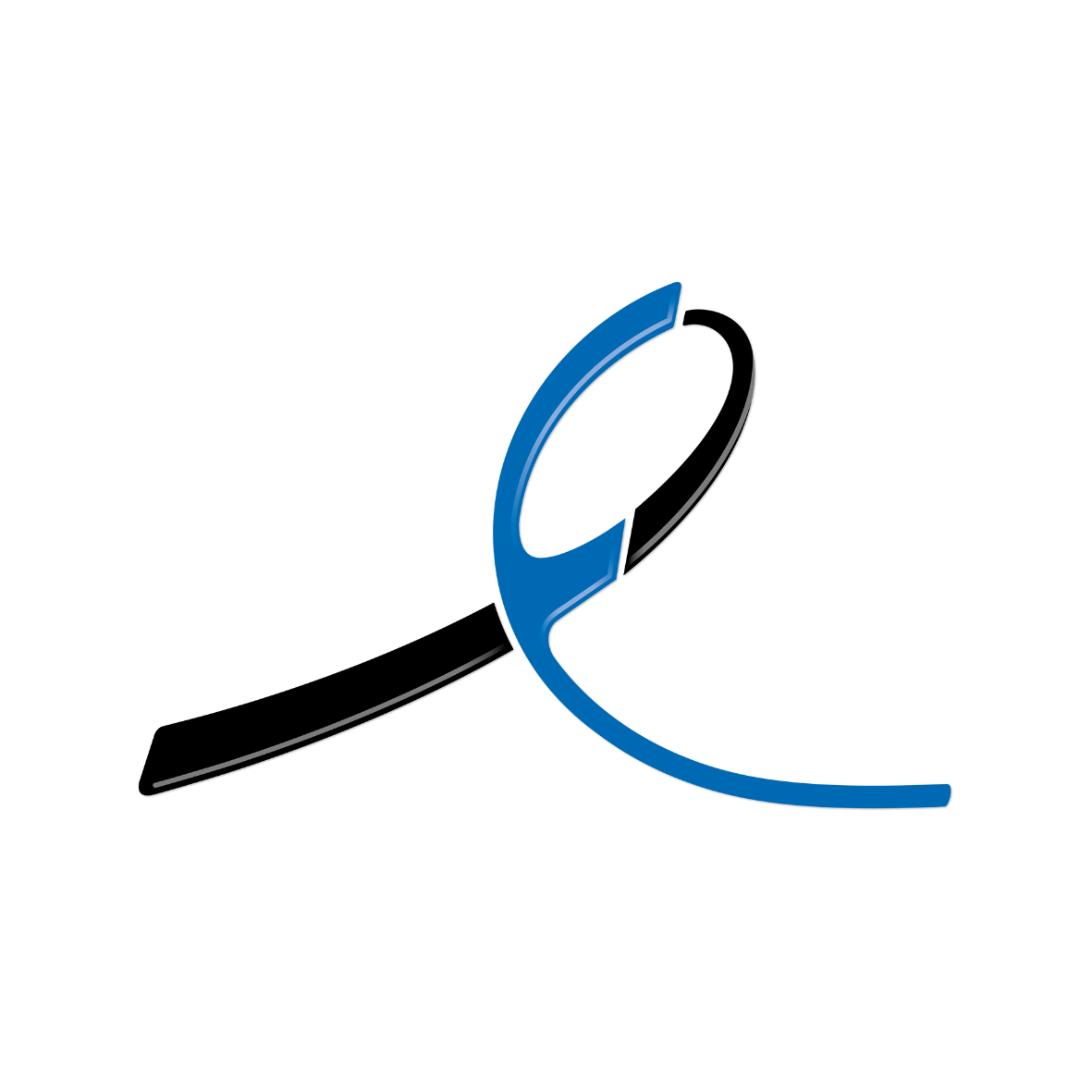
ELvation Marketing Team
Combining sales and flexible customer support with many years’ in-depth knowledge of medical equipment we offer customized solutions to create value with long-term investments and medical supplies. ELvation’s strength lies in its ability to combine the apparently contradictory needs of improving the standards of patient care by providing high-quality medical technology and good corporate profitability. We have a special partnership with Richard Wolf GmbH as their long-term authorized Sales & Service Team for piezo shockwave systems.